This year we give thanks for one of the very few clues we have to the quantum nature of spacetime: black hole entropy. (We’ve previously given thanks for the Standard Model Lagrangian, Hubble’s Law, the Spin-Statistics Theorem, conservation of momentum, effective field theory, the error bar, gauge symmetry, Landauer’s Principle, the Fourier Transform, Riemannian Geometry, the speed of light, the Jarzynski equality, the moons of Jupiter, and space.)
Black holes are regions of spacetime where, according to the rules of Einstein’s theory of general relativity, the curvature of spacetime is so dramatic that light itself cannot escape. Physical objects (those that move at or more slowly than the speed of light) can pass through the “event horizon” that defines the boundary of the black hole, but they never escape back to the outside world. Black holes are therefore black — even light cannot escape — thus the name. At least that would be the story according to classical physics, of which general relativity is a part. Adding quantum ideas to the game changes things in important ways. But we have to be a bit vague — “adding quantum ideas to the game” rather than “considering the true quantum description of the system” — because physicists don’t yet have a fully satisfactory theory that includes both quantum mechanics and gravity.
The story goes that in the early 1970’s, James Bardeen, Brandon Carter, and Stephen Hawking pointed out an analogy between the behavior of black holes and the laws of good old thermodynamics. For example, the Second Law of Thermodynamics (“Entropy never decreases in closed systems”) was analogous to Hawking’s “area theorem”: in a collection of black holes, the total area of their event horizons never decreases over time. Jacob Bekenstein, who at the time was a graduate student working under John Wheeler at Princeton, proposed to take this analogy more seriously than the original authors had in mind. He suggested that the area of a black hole’s event horizon really is its entropy, or at least proportional to it.
This annoyed Hawking, who set out to prove Bekenstein wrong. After all, if black holes have entropy then they should also have a temperature, and objects with nonzero temperatures give off blackbody radiation, but we all know that black holes are black. But he ended up actually proving Bekenstein right; black holes do have entropy, and temperature, and they even give off radiation. We now refer to the entropy of a black hole as the “Bekenstein-Hawking entropy.” (It is just a useful coincidence that the two gentlemen’s initials, “BH,” can also stand for “black hole.”)
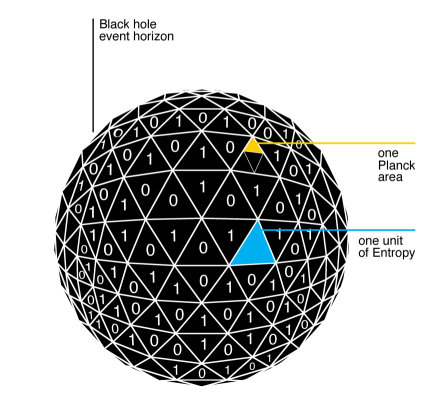
Consider a black hole whose area of its event horizon is . Then its Bekenstein-Hawking entropy is






But there exists a prior puzzle: what is the black hole entropy, anyway? What physical quantity does it describe?
Entropy itself was invented as part of the development of thermodynamics is the mid-19th century, as a way to quantify the transformation of energy from a potentially useful form (like fuel, or a coiled spring) into useless heat, dissipated into the environment. It was what we might call a “phenomenological” notion, defined in terms of macroscopically observable quantities like heat and temperature, without any more fundamental basis in a microscopic theory. But more fundamental definitions came soon thereafter, once people like Maxwell and Boltzmann and Gibbs started to develop statistical mechanics, and showed that the laws of thermodynamics could be derived from more basic ideas of atoms and molecules.
Hawking’s derivation of black hole entropy was in the phenomenological vein. He showed that black holes give off radiation at a certain temperature, and then used the standard thermodynamic relations between entropy, energy, and temperature to derive his entropy formula. But this leaves us without any definite idea of what the entropy actually represents.
One of the reasons why entropy is thought of as a confusing concept is because there is more than one notion that goes under the same name. To dramatically over-simplify the situation, let’s consider three different ways of relating entropy to microscopic physics, named after three famous physicists:
- Boltzmann entropy says that we take a system with many small parts, and divide all the possible states of that system into “macrostates,” so that two “microstates” are in the same macrostate if they are macroscopically indistinguishable to us. Then the entropy is just (the logarithm of) the number of microstates in whatever macrostate the system is in.
- Gibbs entropy is a measure of our lack of knowledge. We imagine that we describe the system in terms of a probability distribution of what microscopic states it might be in. High entropy is when that distribution is very spread-out, and low entropy is when it is highly peaked around some particular state.
- von Neumann entropy is a purely quantum-mechanical notion. Given some quantum system, the von Neumann entropy measures how much entanglement there is between that system and the rest of the world.
These seem like very different things, but there are formulas that relate them to each other in the appropriate circumstances. The common feature is that we imagine a system has a lot of microscopic “degrees of freedom” (jargon for “things that can happen”), which can be in one of a large number of states, but we are describing it in some kind of macroscopic coarse-grained way, rather than knowing what its exact state actually is. The Boltzmann and Gibbs entropies worry people because they seem to be subjective, requiring either some seemingly arbitrary carving of state space into macrostates, or an explicit reference to our personal state of knowledge. The von Neumann entropy is at least an objective fact about the system. You can relate it to the others by analogizing the wave function of a system to a classical microstate. Because of entanglement, a quantum subsystem generally cannot be described by a single wave function; the von Neumann entropy measures (roughly) how many different quantum must be involved to account for its entanglement with the outside world.
So which, if any, of these is the black hole entropy? To be honest, we’re not sure. Most of us think the black hole entropy is a kind of von Neumann entropy, but the details aren’t settled.
One clue we have is that the black hole entropy is proportional to the area of the event horizon. For a while this was thought of as a big, surprising thing, since for something like a box of gas, the entropy is proportional to its total volume, not the area of its boundary. But people gradually caught on that there was never any reason to think of black holes like boxes of gas. In quantum field theory, regions of space have a nonzero von Neumann entropy even in empty space, because modes of quantum fields inside the region are entangled with those outside. The good news is that this entropy is (often, approximately) proportional to the area of the region, for the simple reason that field modes near one side of the boundary are highly entangled with modes just on the other side, and not very entangled with modes far away. So maybe the black hole entropy is just like the entanglement entropy of a region of empty space?
Would that it were so easy. Two things stand in the way. First, Bekenstein noticed another important feature of black holes: not only do they have entropy, but they have the most entropy that you can fit into a region of a fixed size (the Bekenstein bound). That’s very different from the entanglement entropy of a region of empty space in quantum field theory, where it is easy to imagine increasing the entropy by creating extra entanglement between degrees of freedom deep in the interior and those far away. So we’re back to being puzzled about why the black hole entropy is proportional to the area of the event horizon, if it’s the most entropy a region can have. That’s the kind of reasoning that leads to the holographic principle, which imagines that we can think of all the degrees of freedom inside the black hole as “really” living on the boundary, rather than being uniformly distributed inside. (There is a classical manifestation of this philosophy in the membrane paradigm for black hole astrophysics.)
The second obstacle to simply interpreting black hole entropy as entanglement entropy of quantum fields is the simple fact that it’s a finite number. While the quantum-field-theory entanglement entropy is proportional to the area of the boundary of a region, the constant of proportionality is infinity, because there are an infinite number of quantum field modes. So why isn’t the entropy of a black hole equal to infinity? Maybe we should think of the black hole entropy as measuring the amount of entanglement over and above that of the vacuum (called the Casini entropy). Maybe, but then if we remember Bekenstein’s argument that black holes have the most entropy we can attribute to a region, all that infinite amount of entropy that we are ignoring is literally inaccessible to us. It might as well not be there at all. It’s that kind of reasoning that leads some of us to bite the bullet and suggest that the number of quantum degrees of freedom in spacetime is actually a finite number, rather than the infinite number that would naively be implied by conventional non-gravitational quantum field theory.
So — mysteries remain! But it’s not as if we haven’t learned anything. The very fact that black holes have entropy of some kind implies that we can think of them as collections of microscopic degrees of freedom of some sort. (In string theory, in certain special circumstances, you can even identify what those degrees of freedom are.) That’s an enormous change from the way we would think about them in classical (non-quantum) general relativity. Black holes are supposed to be completely featureless (they “have no hair,” another idea of Bekenstein’s), with nothing going on inside them once they’ve formed and settled down. Quantum mechanics is telling us otherwise. We haven’t fully absorbed the implications, but this is surely a clue about the ultimate quantum nature of spacetime itself. Such clues are hard to come by, so for that we should be thankful.
Thanks
Always mind bending and fun to read
Quantum Santa likes it in another world
Lovely post! You have a thorough yet concise way of conveying complex information .
Excellent summary, and beautifully described (for us laymen).
I would also say that I am thankful that there are still many mysteries yet to explore, and so many curious and brilliant minds here to explore them.
Thank you!
Woah. There’s a limit to the “density” of entropy in any single region of our universe, and that limit is found on the surface of a black hole?
I find myself confused, as it would seem to apply that the “end” of the universe, after all black holes have evaporated, will have an entropy *lower* than this limit. Or does this simply represent the entropy of the “quantum foam” of an otherwise empty universe?
@Bobc
No; even though the black hole is at maximum entropy, it evaporates into a universe that is not, so the total entropy still increases.
In classical stat-mech, the entropy of a finite system is a probabilistic thing. But I’ve read that a black hole has the maximum possible entropy for its size (mass, radius, whatever…). If I think of sprinkling 1s and 0s over the diagram of a black hole that you show. There’s only a probabilistic sense in which that can be maximum. For example I can’t (I think) actually infer that the number of 1s and 0s is exactly equal; because that macroscopic bound is the only one consistent with entropy being maximum…can I?
What embodies this information? Information appears to have the same qualities as energy – it needs something to convey it. But perhaps I’m misquided.
The clues seem to suggest, that is just conjecture, that the universe has a finite resolution in some sense both in speed and in density. If you move too fast the degrees of freedom get used up for moving and there aren’t any left for time. If you put too many degrees of freedom close together the universe curves to try to represent it and eventually hits a limit of what the substrate can do. You’re looking at pixels of the hologram, so to speak, and that’s what we think of as the event horizon.
That intuition seems inconsistent with the idea that nothing fancy happens at the event horizon, that you fall in and the real action is at the singularity or that some strange duality allows you to fall in and get crushed into data at the horizon simultaneously. I suspect the latter will turn out to be more correct, and we’ll find the singularity and the event horizon are deformed versions of the same thing. Both are the limit of what the universe can represent, and they appear deformed to different sizes and times to different observers.
Thank you Sean for sharing all these ideas with the public! Folks like me may be talking nonsense, but gosh isn’t the universe the most exciting and profound thing to be talking nonsense about.
What does the Firewall issue mean?
As QFT isn’t Background independent, would this effect how we view entropy of a BH?
Does it mean there’s some part of Quantum Mechanics that’s still an approximation.
12-28-2020
Hi Professor Carroll,
Many thanks. Great information.
Please let us know additionally on these topics.
Obrigada, Sean Carroll.
Sempre um prazer ler suas postages. Aprendo, sempre.
Apenas uma questao: grato(s) a Deus??!!🙄😊
“Black holes are supposed to be completely featureless (they “have no hair,” another idea of Bekenstein’s), with nothing going on inside them once they’ve formed and settled down.”
They seem to have “no hair” according to two independent observations [ https://astronomynow.com/2019/09/12/black-holes-gravitational-ringing-supports-no-hair-theorem/ , https://www.bbc.com/news/science-environment-52464250 ].
“physicists don’t yet have a fully satisfactory theory that includes both quantum mechanics and gravity.”
But with slow roll inflation [Planck collaboration 2018] we could satisfactorily work on all observations with linearized gravity quantum field theory [ http://www.scholarpedia.org/article/Quantum_gravity_as_a_low_energy_effective_field_theory ]. As astrophysicist Ethan Siegel said today in Forbes – something which I tentatively concluded as well: “… in fact [space, time, and the laws of physics] may be [eternal]. Any theorems or proofs to the contrary rely on assumptions whose validity is not soundly established under the conditions which we’d seek to apply them.” So potentially no Planck scale energy density singularities for us.
I’m sure someone can come up with a simpler way to thus consistently model black hole innards than GEODEs demand for another vacuum.
Oops! Forbes article: https://www.forbes.com/sites/startswithabang/2020/11/27/ask-ethan-how-did-the-entire-universe-come-from-nothing/?sh=35c047112c59 .
@ Kevin Casey: “Information appears to have the same qualities as energy – it needs something to convey it.”
Like entropy, information is relative to a system. Quantum information is AFAIK the conservation of likelihoods (unitary) as is seen in say S matrix formulations [ https://en.wikipedia.org/wiki/S-matrix_theory ] – e.g. black holes must “remember” sum of (say) charges.
@Rob: “What does the Firewall issue mean?”
I was asking the same thing! But as I remembered it firewalls preserve BH entropy – and it seems I was right [ https://arxiv.org/pdf/1207.3123.pdf ]. They do so by preventing forbidden quantum state “cloning”.
“As QFT isn’t Background independent, would this effect how we view entropy of a BH?
Does it mean there’s some part of Quantum Mechanics that’s still an approximation.”
If space is on average cosmologically flat, I wouldn’t worry about a classical background [see e.g. my first comment that space may be eternal]. Curvature may just be a simplifying mathematical fiction of tensor fields like field lines is it for vector fields. The Core Theory of Wilczek that Sean has promoted [ https://www.preposterousuniverse.com/blog/2013/01/04/the-world-of-everyday-experience-in-one-equation/ ] explicitly separates out the Lorentz signature metric of relativity as a factor and gravity as an additive effect for quantum path integrals of action.
I don’t think there is something missing in quantum physics – it works and experiments tell us there is nothing hidden. We may not even have to worry about “wavefunction collapse” since it seems it can be interpreted as a relativistic effect of non-preferred reference frames akin to how we not worry about “time dilation” and “length contraction” [ https://www.nature.com/articles/s41598-020-72817-7 ] – non-local spin entanglement experiments and Einstein’s inversion from asking for mechanisms to stating laws [here the conservation of Planck’s constant] can come to the rescue.
What instead comes out as an approximation is AFAIK our scale dependent use of quantum physics, e.g. renormalization theory [ https://en.wikipedia.org/wiki/Renormalization ].
I assume that the universe started with zero or very low entropy which has been increasing ever since. Then, according to the von Neumann interpretation, it must have been initially unentangled. But according to inflation theory, the universe was in causal contact prior to inflation, in which case it should have been highly entangled with high entropy. Can someone untangle me on this?
Your Tday message was my first encounter with your annual gesture. What a great message for us science lovers! I’m thankful for people like you who give thanks to science! I think part of the mess we have found ourselves in in the last four years is because fewer people want to learn the scientific basis for so many important worldly problems and solutions; although, as we know from history, many of the early scientists became that way because they were affluent; democracies still have not perfected a way to bring more people into affluence, so that they can take the time to learn the enlightenment that science offers; thank goodness we will now have a President that again listens to science facts and leaders! I will try and listen to your other Tday science essays!
@ Bruce Fulford
We don’t need cosmic inflation to tell us that the early universe was at thermal equilibrium; that’s a fact obtained from observations of the CMB. In many ways cosmic inflation was invented precisely to make sense of those observations.
So, how can a state of maximal entropy at the same time be a state of minimal entropy?
This is because the early universe is expanding, and when it expands, it cools, and the maximum allowed entropy increases, just like steam in a pressure cooker is at an equilibrium inside the cooker, but very much not at equilibrium with the outside. Further, when at the inception all the fundamental forces were unified in a single hypothetical superforce, as the expanding universe cools, the individual forces precipitate out, as it were, which again raises the maximum allowed entropy, with gravity in particular giving rise to an entropy sink in the form of black holes. To a good approximation, the entropy of the universe in its current state is equal to the entropy of its supermassive black holes, and it will be trillions of years into the future before the plain expansion of the universe “catches up”, and these black holes begin to evaporate away.
Roger Penrose has a lot to say on this subject, and is, like our Sean, well worth listening to.
Oh, and by the way…
Sean Carroll, I am grateful that the world has people like you in it.
Grand merci pour ces développements concernant l’entropie et les trous noirs.
Je suis cependant étonné qu’il ne soit jamais fait allusion aux développement du physicien Jean Charon qui a développé une approche à 8 dimensions (théorie de la relativité complexe) qui répond à bien des questions concernant l’entropie, les trous noirs et l’évolution de l’univers.
Grand merci si nous pouvons échanger, malgré le problème de langue.
As Bruce Fulford says: “‘our’ Sean”; You gotta love it, Sean; we possess you like our “Personal Physicist”, to borrow a phrase from Neil De Grasse Tyson!
If I was American, at next Thanksgiving I would give thanks for Sean Carroll: for his books, blog, podcasts and YouTube lectures and for the generosity of spririt with which he provides them.
Just want to point out that the formula for the Bekenstein-Hawking entropy needs Boltzman’s constant, otherwise it does not make dimensional sense (and Boltzman has to be in there somewhere!).
I have a question with regards to cause and effect.
Assumptions:
Cause = Big Bang
Effect = Everything that happens after the big bang
Exception = life can create a new cause.
Question : If the universe created all the “cause” at its inception (big bang), then how can life / humans create cause outside of what was created in the big bang?
Christopher, I would say that life and intelligence are not externalities impinging on the universe, but rather natural expressions of essence just as space and matter and energy are all aspects of one fundamental essence. It’s all fields, and layers of patterns and constructs emerging therefrom, right? Yet the more we learn the more likely it seems that the essential nature of being, it’s shape and color, could remain hidden from us indefinitely; there may be hard limits to our perception, even considering the potential power of new observational technologies. But I still hope to see humanity succeed as agents of a universe attempting to find itself.